Полипептидные компоненты яда пауков, модулирующие активность потенциал-зависимых натриевых каналов
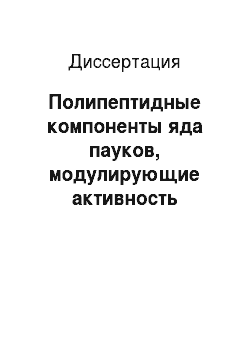
На основании моделей пространственной организации выделенных полипептидов и их сравнения с другими известными токсинами сделано предположение о локализации функционально важных аминокислотных остатков. У р/8-агатоксинов и родственных полипептидов выявлены аминокислотные остатки, вероятно отвечающие за проявление эффектов на активацию и инактивацию каналов. Из яда паука Непаеш те1Шее1 получены три… Читать ещё >
Содержание
- Список сокращений
- 1. Введение
- 2. Обзор литературы
- 2. 1. Структура и функция Ыа+ каналов
- 2. 2. Состав яда пауков
- 2. 2. 1. Низкомолекулярные компоненты
- 2. 2. 2. Пептидные компоненты
- 2. 2. 2. 1. Линейные пептиды
- 2. 2. 2. 2. Цистеин-содержащие пептиды
- 2. 2. 3. Белковые компоненты
- 2. 3. Фундаментальный и прикладной интерес к ядам пауков
- 2. 4. Фармакология потенциал-зависимых Ыа+ каналов
- 2. 4. 1. Поровые блокаторы
- 2. 4. 2. Модуляторы
- 2. 4. 2. 1. Модуляторы активации
- 2. 4. 2. 2. Модуляторы инактивации
- 2. 4. 2. 3. Модуляторы смешанного действия
Полипептидные компоненты яда пауков, модулирующие активность потенциал-зависимых натриевых каналов (реферат, курсовая, диплом, контрольная)
Потенциал-зависимые натриевые (№+) каналы — интегральные мембранные белки, селективно проводящие ионы Ыа+ и играющие ключевую роль в процессах генерации и проведения возбуждения в нервных клетках. Появление каналов в ходе эволюции животных тесно связано с дифференцировкой тканей и обособлением нервной системы. Задействованные в таких важнейших физиологических процессах, как осязание, чувство боли, сокращение мышц, биение сердца и многие другие, каналы представляют большой интерес для ученых всего мира, и с каждым годом актуальность их изучения только возрастает. Несмотря на усилия многих научных коллективов, пространственная структура и механизм работы каналов недостаточно изучены. Поэтому большой фундаментальный интерес представляет задача выяснить молекулярные аспекты механизма ионной проводимости и пространственную организацию этих белков.
В значительной мере современные исследования в области каналов направлены на получение новых лекарств и пестицидов, селективно взаимодействующих с каналами и модифицирующих их активность. Имея в своем распоряжении подобные молекулы, можно направленно управлять работой каналов или, например, корректировать их функционирование в патологических процессах. Поэтому поиск новых лигандов, специфичных для каналов, является важной задачей современной медицины и биотехнологии. каналы являются мишенью для многих компонентов полипептидной природы, выделенных из различных ядовитых животных: анемон, моллюсков, скорпионов, пауков и др. Именно специфичное высокоаффинное взаимодействие с некоторыми лигандами позволило идентифицировать, очистить и охарактеризовать эти мембранные белки.
С точки зрения источника новых модуляторов ионных каналов природные яды представляют наибольший интерес, поскольку являются своеобразными комбинаторными библиотеками биологически активных соединений, отобранных в ходе эволюции. Особое место среди природных ядов занимает яд пауков (в среднем, в яде паука содержится от нескольких десятков до нескольких сотен компонентов), а наиболее интересными оказываются полипептидные компоненты яда, так как они характеризуются высокой специфичностью действия. Благодаря своим свойствам эти соединения получили широкое распространение как инструменты для биохимических исследований. Использование новых лигандов поможет установить точный молекулярный механизм функционирования таких сложных мембранных структур, как Ыа+ каналы, а также пролить свет на их фармакологические характеристики и пространственную организацию. Новые инструменты могут быть полезны в фундаментальных исследованиях молекулярных механизмов нейросигнализации, а так же найдут практическое применение в медицине для терапии заболеваний, связанных с дисфункцией каналов, и в биотехнологии как безопасные биоинсектициды.
Цель и задачи работы.
Цель данной работы — поиск и идентификация новых полипептидных веществ из яда пауков, модулирующих активность Ыа+ каналов. В соответствии с целью были поставлены следующие конкретные задачи:
1. Провести тестирование коллекции ядов пауков в отношении каналов.
2. Выделить и установить первичную структуру активных полипептидов.
3. Изучить механизм действия выделенных компонентов.
4. Осуществить поиск структурных детерминант активности новых соединений.
2. ОБЗОР ЛИТЕРАТУРЫ.
5. ВЫВОДЫ:
1. Проведено тестирование коллекции ядов 16 видов пауков из 9 семейств с целью поиска модуляторов функциональной активности потенциал-зависимых каналов насекомых и млекопитающих. Показано, что яд Непаеш те1Ыее1 обладает наибольшим ингибирующим действием на каналы млекопитающих, а яд Agelena опеШаШ высокотоксичен для насекомых.
2. Из яда паука Непаеш те1Шее1 получены три полипептида (Нт-1−3), относящиеся к новым группам блокаторов Ыа+ каналов млекопитающих и насекомых. Эти полипептиды длиной 35−40 остатков содержат по 6 остатков цистеина, образующих 3 внутримолекулярные дисульфидные связи. Согласно полученным данным, Нт-1 и 2 являются поровыми блокаторами, а Нш-3 — ингибитором активации каналов.
3. Из яда паука Agelena опеЫаШ получены семь полипептидов (|3/5-агатоксины-1−7), специфично воздействующих на каналы насекомых. Все они содержат 36−38 остатков, среди которых 8 являются остатками цистеина, образующих 4 внутримолекулярные дисульфидные связи. Уникальность р/5-агатоксинов заключается в их способности к одновременному и потенциал-зависимому действию на процессы активации и инактивации каналов.
4. На основании моделей пространственной организации выделенных полипептидов и их сравнения с другими известными токсинами сделано предположение о локализации функционально важных аминокислотных остатков. У р/8-агатоксинов и родственных полипептидов выявлены аминокислотные остатки, вероятно отвечающие за проявление эффектов на активацию и инактивацию каналов.
6. БЛАГОДАРНОСТИ.
Автор выражает свою признательность:
— Коллективу лаборатории нейрорецепторов и нейрорегуляторов ИБХ РАН и лично Егорову Ц. А. и Мусолямову А. Х. за помощь при секвенировании пептидовВасилевскому A.A. за ценнейшие указания и помощь в написании научных статей, Кузьменкову А. И. за помощь в подготовке рисунков.
— Коллективу лаборатории токсикологии Католического Университета города Левен, Бельгия и лично Б. Биллену и Я. Титгату за проведение экспериментов по выяснению биологической активности токсинов и их механизма действия, С. Дебавье за приготовление мРНК и выделение ооцитов.
— Коллективу лаборатории молекулярной токсикологии ИБХ РАН и лично Филькину С. Ю. за помощь в построении пространственных моделей пептидов.
— Федорову A.A. (ТОО «Научно-производственное объединение Фауна" — Алматы, Республика Казахстан) за предоставленные образцы яда пауков.
4.7, Заключение.
Структурное разнообразие и важная роль Ыа" каналов во многих биологических процессах обуславливает интерес к этим объектам со стороны исследователей. Неудивительно, что в настоящее время описано большое количество лигандов Ыа каналов различной природы. Представленная работа дополняет существующее многообразие, демонстрируя, как с помощью арсенала современных методов в яде пауков можно найти и охарактеризовать вещества, проявляющие активность в отношении Ыа' каналов млекопитающих и насекомых. Обнаруженные полипептиды являются как поровыми блокаторами, так и модуляторами различных стадий работы каналов — активации и инактивации. Кроме того, некоторые из найденных токсинов проявляют высокую специфичность в отношении насекомых и могут быть использованы как основа для разработки эффективных и безопасных инсектицидов.
Список литературы
- Catterall WA (2000) From ionic currents to molecular mechanisms: the structure and function of voltage-gated sodium channels. Neuron 26, 13−25.
- Hille B (2001) Ion Channels of Excitable Membranes 3rd edn. Sinauer Associates.
- Yu FH & Catterall WA (2003) Overview of the voltage-gated sodium channel family. Genome Biol 4, 207.
- Chahine M & O’Leary ME (2011) Regulatory role of voltage-gated Na channel beta subunits in sensory neurons. Front Pharmacol 2, 70.
- Schreibmayer W, Wallner M & Lotan I (1994) Mechanism of modulation of single sodium channels from skeletal muscle by the beta 1-subunit from rat brain. Pflugers Arch 426, 360−362.
- Isom LL, De Jongh KS, Patton DE, Reber BF, Offord J, Charbonneau H, Walsh K, Goldin AL & Catterall WA (1992) Primary structure and functional expression of the beta 1 subunit of the rat brain sodium channel. Science 256, 839 842.
- Isom LL, Ragsdale DS, De Jongh KS, Westenbroek RE, Reber BF, Scheuer T & Catterall WA (1995) Structure and function of the beta 2 subunit of brain sodium channels, a transmembrane glycoprotein with a CAM motif. Cell 83, 433 442.
- Johnson D, Montpetit ML, Stocker PJ & Bennett ES (2004) The sialic acid component of the betal subunit modulates voltage-gated sodium channel function. J Biol Chem 279, 44 303−44 310.
- Bennett ES (2002) Isoform-specific effects of sialic acid on voltage-dependent Na+ channel gating: functional sialic acids are localized to the S5-S6 loop of domain I. J Physiol 538, 675−690.
- Furini S & Domene C (2011) Gating at the selectivity filter of ion channels that conduct Na+ and K+ ions. Biophys J101, 1623−1631.
- Lipkind GM & Fozzard HA (2000) KcsA crystal structure as framework for a molecular model of the Na (+) channel pore. Biochemistry 39, 8161−8170.
- Yang N, George AL, Jr. & Horn R (1996) Molecular basis of charge movement in voltage-gated sodium channels. Neuron 16, 113−122.
- McCollum IJ, Vilin YY, Spackman E, Fujimoto E & Ruben PC (2003) Negatively charged residues adjacent to IFM motif in the DIII-DIV linker of hNa (V)1.4 differentially affect slow inactivation. FEBSLett 552, 163−169.
- West JW, Patton DE, Scheuer T, Wang Y, Goldin AL & Catterall WA (1992) A cluster of hydrophobic amino acid residues required for fast Na (+)-channel inactivation. Proc Natl Acad Sci USA 89, 10 910−10 914.
- Miyamoto K, Kanaori K, Nakagawa T & Kuroda Y (2001) Solution structures of the inactivation gate particle peptides of rat brain type-IIA and human heart sodium channels in SDS micelles. JPept Res 57, 203−214.
- Febvre-Chevalier C, Bilbaut A, Bone Q & Febvre J (1985) Sodium-calcium action potential associated with contraction in the heliozoan Actinocoryne contractilis. J Exp Biol 122, 177−192.
- Lopreato GF, Lu Y, Southwell A, Atkinson NS, Hillis DM, Wilcox TP & Zakon HH (2001) Evolution and divergence of sodium channel genes in vertebrates. Proc Natl Acad Sci USA 98, 7588−7592.
- Catterall WA, Goldin AL & Waxman SG (2005) International Union of Pharmacology. XLVII. Nomenclature and structure-function relationships of voltage-gated sodium channels. Pharmacol Rev 57, 397−409.
- Tan J, Liu Z, Nomura Y, Goldin AL & Dong K (2002) Alternative splicing of an insect sodium channel gene generates pharmacologically distinct sodium channels. JNeurosci 22, 5300−5309.
- Dong K (2007) Insect sodium channels and insecticide resistance. Invert Neurosci 7, 17−30.
- Goldin AL, Barchi RL, Caldwell JH, Hofmann F, Howe JR, Hunter JC, Kallen RG, Mandel G, Meisler MH, Netter YB, et al. (2000) Nomenclature of voltage-gated sodium channels. Neuron 28, 365−368.
- Goldin AL (1999) Diversity of mammalian voltage-gated sodium channels. Ann N YAcadSci 868, 38−50.
- Yamaoka K, Vogel SM & Seyama I (2006) Na+ channel pharmacology and molecular mechanisms of gating. Curr Pharm Des 12, 429−442.
- Lehmann-Horn F & Jurkat-Rott K (1999) Voltage-gated ion channels and hereditary disease. Physiol Rev 79, 1317−1372.
- Cannon SC (2006) Pathomechanisms in channelopathies of skeletal muscle and brain. Annu Rev Neurosci 29, 387−415.
- Scalmani P, Rusconi R, Armatura E, Zara F, Avanzini G, Franceschetti S & Mantegazza M (2006) Effects in neocortical neurons of mutations of the Na (v)1.2 Na+ channel causing benign familial neonatal-infantile seizures. J Neurosci 26, 10 100−10 109.
- Meisler MH, O’Brien JE & Sharkey LM (2010) Sodium channel gene family: epilepsy mutations, gene interactions and modifier effects. J Physiol 588, 1841−1848.
- Amin AS, Asghari-Roodsari A & Tan HL (2010) Cardiac sodium channelopathies. Pflugers Arch 460, 223−237.
- Cannon SC (2010) Voltage-sensor mutations in channelopathies of skeletal muscle. J Physiol 588, 1887−1895.
- Andavan GS & Lemmens-Gruber R (2011) Voltage-gated sodium channels: mutations, channelopathies and targets. Curr Med Chem 18, 377−397.
- Escayg A & Goldin AL (2010) Sodium channel SCN1A and epilepsy: mutations and mechanisms. Epilepsia 51, 1650−1658.
- Sato С, Ueno Y, Asai K, Takahashi K, Sato M, Engel A & Fujiyoshi Y (2001) The voltage-sensitive sodium channel is a bell-shaped molecule with several cavities. Nature 409, 1047−1051.
- Long SB, Campbell EB & Mackinnon R (2005) Crystal structure of a mammalian voltage-dependent Shaker family K+ channel. Science 309, 897−903.
- Payandeh J, Scheuer T, Zheng N & Catterall WA (2011) The crystal structure of a voltage-gated sodium channel. Nature 475, 353−358.
- Koishi R, Xu H, Ren D, Navarro B, Spiller BW, Shi Q & Clapham DE (2004) A superfamily of voltage-gated sodium channels in bacteria. J Biol Chem 279, 9532−9538.
- Zhao Y, Scheuer T & Catterall WA (2004) Reversed voltage-dependent gating of a bacterial sodium channel with proline substitutions in the S6 transmembrane segment. Proc Natl Acad Sci USA 101, 17 873−17 878.
- Yue L, Navarro B, Ren D, Ramos A & Clapham DE (2002) The cation selectivity filter of the bacterial sodium channel, NaChBac. J Gen Physiol 120, 845−853.
- Long SB, Tao X, Campbell EB & MacKinnon R (2007) Atomic structure of a voltage-dependent K+ channel in a lipid membrane-like environment. Nature 450, 376−382.
- Fozzard HA, Lee PJ & Lipkind GM (2005) Mechanism of local anesthetic drug action on voltage-gated sodium channels. Curr Pharm Des 11, 2671−2686.
- Platnick N1 (1997) Advances in spider taxonomy. New York Entomological Society and The American Museum of Natural History, (updated online version available at http://researchamnhorg/entomology/spiders/catalog81−87/).
- Василевский А. КС, Гришин E. (2009) Молекулярное разнообразие яда пауков. Успехи биологической химии 49, 211−274.
- Wigger Е, Kuhn-Nentwig L & Nentwig W (2002) The venom optimisation hypothesis: a spider injects large venom quantities only into difficult prey types. Toxicon 40, 749−752.
- Malli H, Kuhn-Nentwig L, Imboden H & Nentwig W (1999) Effects of size, motility and paralysation time of prey on the quantity of venom injected by the hunting spider Cupiennius salei. J Exp Biol 202, 2083−2089.
- Vapenik Z & Nentwig W (2000) The influence of hunger and breeding temperature on the venom production of the spider Cupiennius salei (Araneae, Ctenidae). Toxicon 38, 293−298.
- Шляпников ЮМ, Козлов CA, Федоров AA & Гришин ЕВ (2010) Сравнение полипептидного состава яда индивидуальных пауков Agelena orientalis. Биоорг химия 36, 81−88.
- Rash LD & Hodgson WC (2002) Pharmacology and biochemistry of spider venoms. Toxicon 40, 225−254.
- Corzo G & Escoubas P (2003) Pharmacologically active spider peptide toxins. Cell Mol Life Sci 60, 2409−2426.49. da Silva PH, da Silveira RB, Appel MH, Mangili ОС, Gremski W & Veiga SS (2004) Brown spiders and loxoscelism. Toxicon 44, 693−709.
- Binford GJ & Wells MA (2003) The phylogenetic distribution of sphingomyelinase D activity in venoms of Haplogyne spiders. Comp Biochem Physiol В Biochem Mol Biol 135, 25−33.
- Grishin EV (1998) Black widow spider toxins: the present and the future. Toxicon 36, 1693−1701.
- Vassilevski AA, Kozlov SA, Samsonova OV, Egorova NS, Karpunin DV, Pluzhnikov KA, Feofanov AV & Grishin EV (2008) Cyto-insectotoxins, a novel class of cytolytic and insecticidal peptides from spider venom. Biochem J 411, 687−696.
- Гришин ЕВ, Волкова TM & Арсеньев АС (1988) Антагонисты глутаматных рецепторов из яда паука Argiope lobata. Биоорг химия 14, 883 892.
- Wullschleger В, Nentwig W & Kuhn-Nentwig L (2005) Spider venom: enhancement of venom efficacy mediated by different synergistic strategies in Cupiennius salei. J Exp Biol 208, 2115−2121.
- Mebs D (2001) Toxicity in animals. Trends in evolution? Toxicon 39, 87−96.
- Escoubas P & King GF (2009) Venomics as a drug discovery platform. Expert Rev Proteomics 6, 221−224.
- Kuhn-Nentwig L, Schaller J & Nentwig W (2004) Biochemistry, toxicology and ecology of the venom of the spider Cupiennius salei (Ctenidae). Toxicon 43, 543−553.
- Rash LD, King RG & Hodgson WC (1998) Evidence that histamine is the principal pharmacological component of venom from an Australian wolf spider (Lycosa godeffroyi). Toxicon 36, 367−375.
- Meinwald J & Eisner T (1995) The chemistry of phyletic dominance. Proc Natl Acad Sci USA 92, 14−18.
- Гришин ЕВ, Волкова TM, Арсеньев АС, Решетова ОС, Оноприенко ВВ, Магазаник ЛГ, Антонов СМ & Федорова ИМ (1986) Структурно-функциональная характеристика аргиопина блокатора ионных каналов из яда паука Argiope lobata. Биоорг химия 12 1121−1124.
- Osborne RH (1996) Insect neurotransmission: neurotransmitters and their receptors. Pharmacol Ther 69, 117−142.
- Estrada G, Villegas E & Corzo G (2007) Spider venoms: a rich source of acylpolyamines and peptides as new leads for CNS drugs. Nat Prod Rep 24, 145 161.
- Grishin E (1999) Polypeptide neurotoxins from spider venoms. Eur J Biochem 264, 276−280.
- Kuhn-Nentwig L (2003) Antimicrobial and cytolytic peptides of venomous arthropods. Cell Mol Life Sci 60, 2651−2668.
- Andreu D & Rivas L (1998) Animal antimicrobial peptides: an overview. Biopolymers 47, 415−433.
- Kuhn-Nentwig L, Muller J, Schaller J, Walz A, Dathe M & Nentwig W (2002) Cupiennin 1, a new family of highly basic antimicrobial peptides in the venom of the spider Cupiennius salei (Ctenidae). J Biol Chem 277,11 208−11 216.
- Budnik BA, Olsen JV, Egorov TA, Anisimova VE, Galkina TG, Musolyamov AK, Grishin EV & Zubarev RA (2004) De novo sequencing of antimicrobial peptides isolated from the venom glands of the wolf spider Lycosa singoriensis. J Mass Spectrom 39, 193−201.
- King GF, Gentz MC, Escoubas P & Nicholson GM (2008) A rational nomenclature for naming peptide toxins from spiders and other venomous animals. Toxicon 52, 264−276.
- Escoubas P (2006) Molecular diversification in spider venoms: a web of combinatorial peptide libraries. Mol Divers 10, 545−554.
- Craik DJ, Daly NL & Waine C (2001) The cystine knot motif in toxins and implications for drug design. Toxicon 39, 43−60.
- Norton RS & Pallaghy PK (1998) The cystine knot structure of ion channel toxins and related polypeptides. Toxicon 36, 1573−1583.
- Catterall WA, Cestele S, Yarov-Yarovoy V, Yu FH, Konoki K & Scheuer T (2007) Voltage-gated ion channels and gating modifier toxins. Toxicon 49, 124 141.
- King GF (2007) Modulation of insect Ca (v) channels by peptidic spider toxins. Toxicon 49, 513−530.
- Kozlov S & Grishin E (2005) Classification of spider neurotoxins using structural motifs by primary structure features. Single residue distribution analysis and pattern analysis techniques. Toxicon 46, 672−686.
- Shikata Y, Watanabe T, Teramoto T, Inoue A, Kawakami Y, Nishizawa Y, Katayama K & Kuwada M (1995) Isolation and characterization of a peptide isomerase from funnel web spider venom. J Biol Chem 270, 16 719−16 723.
- Lee S & Lynch KR (2005) Brown recluse spider (Loxosceles reclusa) venom phospholipase D (PLD) generates lysophosphatidic acid (LPA). Biochem J 391,317−323.
- Ushkaryov YA, Rohou A & Sugita S (2008) alpha-Latrotoxin and its receptors. Handb Exp Pharmacol, 171−206.
- Rohou A, Nield J & Ushkaryov YA (2007) Insecticidal toxins from black widow spider venom. Toxicon 49, 531−549.
- Vassilevski AA, Kozlov SA & Grishin EV (2008) Antimicrobial peptide precursor structures suggest effective production strategies. Recent Pat Inflamm Allergy Drug Discov 2, 58−63.
- Kolmar H (2009) Biological diversity and therapeutic potential of natural and engineered cystine knot miniproteins. Curr Opin Pharmacol 9, 608−614.
- Narahashi T (2008) Tetrodotoxin: a brief history. Proc Jpn Acad Ser B Phys Biol Sci 84, 147−154.
- Tan CT & Lee EJ (1986) Paralytic shellfish poisoning in Singapore. Ann Acad Med Singapore 15, 77−79.
- Araoz R, Molgo J & Tandeau de Marsac N (2010) Neurotoxic cyanobacterial toxins. Toxicon 56, 813−828.
- Santarelli VP, Eastwood AL, Dougherty DA, Horn R & Ahern CA (2007) A cation-pi interaction discriminates among sodium channels that are either sensitive or resistant to tetrodotoxin block. J Biol Chern 282, 8044−8051.
- Terlau H & Olivera BM (2004) Conus venoms: a rich source of novel ion channel-targeted peptides. Physiol Rev 84, 41−68.
- Han TS, Teichert RW, Olivera BM & Bulaj G (2008) Conus venoms a rich source of peptide-based therapeutics. Curr Pharm Des 14, 2462−2479.
- Dudley SC, Jr., Chang N, Hall J, Lipkind G, Fozzard HA & French RJ2000) mu-conotoxin GIIIA interactions with the voltage-gated Na (+) channel predict a clockwise arrangement of the domains. J Gen Physiol 116, 679−690.
- Hill JM, Alewood PF & Craik DJ (1996) Three-dimensional solution structure of mu-conotoxin GIIIB, a specific blocker of skeletal muscle sodium channels. Biochemistry 35, 8824−8835.
- Nakamura M, Niwa Y, Ishida Y, Kohno T, Sato K, Oba Y & Nakamura H2001) Modification of Arg-13 of mu-conotoxin GIIIA with piperidinyl-Arg analogs and their relation to the inhibition of sodium channels. FEBS Lett 503, 107−110.
- Liang S (2004) An overview of peptide toxins from the venom of the Chinese bird spider Selenocosmia huwena Wang =Ornithoctonus huwena (Wang). Toxicon 43, 575−585.
- Li D, Xiao Y, Hu W, Xie J, Bosmans F, Tytgat J & Liang S (2003) Function and solution structure of hainantoxin-I, a novel insect sodium channel inhibitor from the Chinese bird spider Selenocosmia hainana. FEBS Lett 555, 616−622.
- Xiao YC & Liang SP (2003) Purification and characterization of Hainantoxin-V, a tetrodotoxin-sensitive sodium channel inhibitor from the venom of the spider Selenocosmia hainana. Toxicon 41, 643−650.
- Liu Z, Dai J, Chen Z, Hu W, Xiao Y & Liang S (2003) Isolation and characterization of hainantoxin-IV, a novel antagonist of tetrodotoxin-sensitive sodium channels from the Chinese bird spider Selenocosmia hainana. Cell Mol Life SW60, 972−978.
- Xiao Y & Liang S (2003) Inhibition of neuronal tetrodotoxin-sensitive Na+ channels by two spider toxins: hainantoxin-III and hainantoxin-IV. Eur J Pharmacol 477, 1−7.
- Li D, Xiao Y, Xu X, Xiong X, Lu S, Liu Z, Zhu Q, Wang M, Gu X & Liang S (2004) Structure—activity relationships of hainantoxin-IV and structure determination of active and inactive sodium channel blockers. J Biol Chem 279, 37 734−37 740.
- Butterworth JFt & Strichartz GR (1990) Molecular mechanisms of local anesthesia: a review. Anesthesiology 72, 711−734.
- Albuquerque EX, Daly JW & Witkop B (1971) Batrachotoxin: chemistry and pharmacology. Science 172, 995−1002.
- Constantine GH, Jr., Sheth K & Catalfomo P (1967) Grayanotoxin. I. Occurrence in additional Ericaceae species. JPharm Sci 56, 1518−1519.
- Ulbricht W (1969) The effect of veratridine on excitable membranes of nerve and muscle. Ergeb Physiol 61, 18−71.
- Chan TY (2009) Aconite poisoning. Clin Toxicol (Phila) 47, 279−285.
- Wang SY & Wang GK (1998) Point mutations in segment I-S6 render voltage-gated Na+ channels resistant to batrachotoxin. Proc Natl Acad Sci USA 95, 2653−2658.
- Wang SY & Wang GK (1999) Batrachotoxin-resistant Na+ channels derived from point mutations in transmembrane segment D4-S6. Biophys J76, 3141−3149.
- Tikhonov DB & Zhorov BS (2005) Sodium channel activators: model of binding inside the pore and a possible mechanism of action. FEBS Lett 579, 42 074 212.
- Wang SY & Wang GK (2003) Voltage-gated sodium channels as primary targets of diverse lipid-soluble neurotoxins. Cell Signal 15, 151−159.
- Cestele S, Yarov-Yarovoy V, Qu Y, Sampieri F, Scheuer T & Catterall WA (2006) Structure and function of the voltage sensor of sodium channels probed by a beta-scorpion toxin. J Biol Chern 281, 21 332−21 344.
- Gurevitz M, Karbat I, Cohen L, Ilan N, Kahn R, Turkov M, Stankiewicz M, Stuhmer W, Dong K & Gordon D (2007) The insecticidal potential of scorpion beta-toxins. Toxicon 49, 473−489.
- Billen B, Bosmans F & Tytgat J (2008) Animal peptides targeting voltage-activated sodium channels. Curr Pharm Des 14, 2492−2502.
- Cestele S, Qu Y, Rogers JC, Rochat H, Scheuer T & Catterall WA (1998) Voltage sensor-trapping: enhanced activation of sodium channels by beta-scorpion toxin bound to the S3-S4 loop in domain II. Neuron 21, 919−931.
- Cestele S, Scheuer T, Mantegazza M, Rochat H & Catterall WA (2001) Neutralization of gating charges in domain II of the sodium channel alpha subunit enhances voltage-sensor trapping by a beta-scorpion toxin. J Gen Physiol 118, 291−302.
- Martin MF, Garcia y Perez LG, el Ayeb M, Kopeyan C, Bechis G, Jover E & Rochat H (1987) Purification and chemical and biological characterizations of seven toxins from the Mexican scorpion, Centruroides suffusus suffusus. J Biol Chem 262, 4452−4459.
- Kopeyan C, Mansuelle P, Sampieri F, Brando T, Bahraoui EM, Rochat H & Granier C (1990) Primary structure of scorpion anti-insect toxins isolated from the venom of Leiurus quinquestriatus quinquestriatus. FEBS Lett 261, 423−426.
- Froy O, Zilberberg N, Gordon D, Turkov M, Gilles N, Stankiewicz M, Pelhate M, Loret E, Oren DA, Shaanan B, et al. (1999) The putative bioactive surface of insect-selective scorpion excitatory neurotoxins. J Biol Chem 274, 57 695 776.
- Benkhalifa R, Stankiewicz M, Lapied B, Turkov M, Zilberberg N, Gurevitz M & Pelhate M (1997) Refined electrophysiological analysis suggests that a depressant toxin is a sodium channel opener rather than a blocker. Life Sci 61, 819 830.
- Gordon D, Moskowitz H, Eitan M, Warner C, Catterall WA & Zlotkin E (1992) Localization of receptor sites for insect-selective toxins on sodium channels by site-directed antibodies. Biochemistry 31, 7622−7628.
- Bechis G, Sampieri F, Yuan PM, Brando T, Martin MF, Diniz CR & Rochat H (1984) Amino acid sequence of toxin VII, a beta-toxin from the venom of the scorpion Tityus serrulatus. Biochem Biophys Res Commun 122, 1146−1153.
- Gordon D, Ilan N, Zilberberg N, Gilles N, Urbach D, Cohen L, Karbat I, Froy O, Gaathon A, Kallen RG, et al. (2003) An 'Old World' scorpion beta-toxin that recognizes both insect and mammalian sodium channels. Eur J Biochem 270, 2663−2670.
- Skinner WS, Adams ME, Quistad GB, Kataoka H, Cesarin BJ, Enderlin FE & Schooley DA (1989) Purification and characterization of two classes of neurotoxins from the funnel web spider, Agelenopsis aperta. J Biol Chem 264, 2150−2155.
- Adams ME (2004) Agatoxins: ion channel specific toxins from the American funnel web spider, Agelenopsis aperta. Toxicon 43, 509−525.
- Omecinsky DO, Holub KE, Adams ME & Reily MD (1996) Three-dimensional structure analysis of mu-agatoxins: further evidence for common motifs among neurotoxins with diverse ion channel specificities. Biochemistry 35, 2836−2844.
- Stapleton A, Blankenship DT, Ackermann BL, Chen TM, Gorder GW, Manley GD, Palfreyman MG, Coutant JE & Cardin AD (1990) Curtatoxins. Neurotoxic insecticidal polypeptides isolated from the funnel-web spider Hololena curta. J Biol Chem 265, 2054−2059.
- Xiao Y, Tang J, Yang Y, Wang M, Hu W, Xie J, Zeng X & Liang S (2004) Jingzhaotoxin-III, a novel spider toxin inhibiting activation of voltage-gated sodium channel in rat cardiac myocytes. J Biol Chem 279, 26 220−26 226.
- Middleton RE, Warren VA, Kraus RL, Hwang JC, Liu CJ, Dai G, Brochu RM, Kohler MG, Gao YD, Garsky VM, et al. (2002) Two tarantula peptides inhibit activation of multiple sodium channels. Biochemistry 41, 14 734−14 747.
- Priest BT, Blumenthal KM, Smith JJ, Warren VA & Smith MM (2007) ProTx-I and ProTx-II: gating modifiers of voltage-gated sodium channels. Toxicon 49,194−201.
- Xiao Y, Jackson JO, 2nd, Liang S & Cummins TR (2011) Common molecular determinants of tarantula huwentoxin-IV inhibition of Na+ channel voltage sensors in domains II and IV. J Biol Chem 286, 27 301−27 310.
- Leipold E, DeBie H, Zorn S, Borges A, Olivera BM, Terlau H & Heinemann SH (2007) muO conotoxins inhibit NaV channels by interfering with their voltage sensors in domain-2. Channels (Austin) 1, 253−262.
- Zorn S, Leipold E, Hansel A, Bulaj G, Olivera BM, Terlau H & Heinemann SH (2006) The muO-conotoxin MrVIA inhibits voltage-gated sodium channels by associating with domain-3. FEBS Lett 580, 1360−1364.
- Campos FV, Chanda B, Beirao PS & Bezanilla F (2008) Alpha-scorpion toxin impairs a conformational change that leads to fast inactivation of muscle sodium channels. J Gen Physiol 132, 251−263.
- Possani LD, Becerril B, Delepierre M & Tytgat J (1999) Scorpion toxins specific for Na±channels. Eur JBiochem 264, 287−300.
- Rochat H, Rochat C, Sampieri F, Miranda F & Lissitzky S (1972) The amino-acid sequence of neurotoxin II of Androctonus australis Hector. Eur J Biochem 28, 381−388.
- Eitan M, Fowler E, Herrmann R, Duval A, Pelhate M & Zlotkin E (1990) A scorpion venom neurotoxin paralytic to insects that affects sodium current inactivation: purification, primary structure, and mode of action. Biochemistry 29, 5941−5947.
- Kopeyan C, Mansuelle P, Martin-Eauclaire MF, Rochat H & Miranda F (1993) Characterization of toxin III of the scorpion Leiurus quinquestriatus quinquestriatus: a new type of alpha-toxin highly toxic both to mammals and insects. Nat Toxins 1, 308−312.
- Borchani L, Stankiewicz M, Kopeyan C, Mansuelle P, Kharrat R, Cestele S, Karoui H, Rochat H, Pelhate M & el Ayeb M (1997) Purification, structure andactivity of three insect toxins from Buthus occitanus tunetanus venom. Toxicon 35, 365−382.
- Arnon T, Potikha T, Sher D, Elazar M, Mao W, Tal T, Bosmans F, Tytgat J, Ben-Arie N & Zlotkin E (2005) BjalphalT: a novel scorpion alpha-toxin selective for insects—unique pharmacological tool. Insect Biochem Mol Biol 35, 187−195.
- Sautiere P, Cestele S, Kopeyan C, Martinage A, Drobecq H, Doljansky Y & Gordon D (1998) New toxins acting on sodium channels from the scorpion Leiurus quinquestriatus hebraeus suggest a clue to mammalian vs insect selectivity. Toxicon 36, 1141−1154.
- Vargas O, Martin MF & Rochat H (1987) Characterization of six toxins from the venom of the Moroccan scorpion Buthus occitanus mardochei. Eur J Biochem 162, 589−599.
- Goudet C, Huys I, Clynen E, Schoofs L, Wang DC, Waelkens E & Tytgat J (2001) Electrophysiological characterization of BmK Ml, an alpha-like toxin from Buthus martensi Karsch venom. FEBS Lett 495, 61−65.
- Wang CG, Gilles N, Hamon A, Le Gall F, Stankiewicz M, Pelhate M, Xiong YM, Wang DC & Chi CW (2003) Exploration of the functional site of a scorpion alpha-like toxin by site-directed mutagenesis. Biochemistry 42, 4699−4708.
- Liu LH, Bosmans F, Maertens C, Zhu RH, Wang DC & Tytgat J (2005) Molecular basis of the mammalian potency of the scorpion alpha-like toxin, BmK Ml. FASEB J19, 594−596.
- He XL, Li HM, Zeng ZH, Liu XQ, Wang M & Wang DC (1999) Crystal structures of two alpha-like scorpion toxins: non-proline cis peptide bonds and implications for new binding site selectivity on the sodium channel. J Mol Biol 292,125−135.
- Sun YM, Bosmans F, Zhu RH, Goudet C, Xiong YM, Tytgat J & Wang DC (2003) Importance of the conserved aromatic residues in the scorpion alpha-like toxin BmK Ml: the hydrophobic surface region revisited. J Biol Chem 278, 2 412 524 131.
- Li HM, Zhao T, Jin L, Wang M, Zhang Y & Wang DC (1999) A series of bioactivity-variant neurotoxins from scorpion Buthus martensii Karsch: purification, crystallization and crystallographic analysis. Acta Crystallogr D Biol Crystallogr 55, 341−344.
- Li HM, Wang DC, Zeng ZH, Jin L & Hu RQ (1996) Crystal structure of an acidic neurotoxin from scorpion Buthus martensii Karsch at 1.85 A resolution. J Mol Biol 261, 415−431.
- Zilberberg N, Froy O, Loret E, Cestele S, Arad D, Gordon D & Gurevitz M (1997) Identification of structural elements of a scorpion alpha-neurotoxin important for receptor site recognition. J Biol Chem 272, 14 810−14 816.
- Karbat I, Frolow F, Froy O, Gilles N, Cohen L, Turkov M, Gordon D & Gurevitz M (2004) Molecular basis of the high insecticidal potency of scorpion alpha-toxins. J Biol Chem 279, 31 679−31 686.
- Karbat I, Kahn R, Cohen L, Ilan N, Gilles N, Corzo G, Froy O, Gur M, Albrecht G, Heinemann SH, et al. (2007) The unique pharmacology of the scorpion alpha-like toxin Lqh3 is associated with its flexible C-tail. FEBS J 274, 1918−1931.
- Ye X, Bosmans F, Li C, Zhang Y, Wang DC & Tytgat J (2005) Structural basis for the voltage-gated Na+ channel selectivity of the scorpion alpha-like toxin BmKMl. JMolBiol 353, 788−803.
- Nicholson GM, Walsh R, Little MJ & Tyler MI (1998) Characterisation of the effects of robustoxin, the lethal neurotoxin from the Sydney funnel-web spider Atrax robustus, on sodium channel activation and inactivation. Pflugers Arch 436, 117−126.
- Nicholson GM, Willow M, Howden ME & Narahashi T (1994) Modification of sodium channel gating and kinetics by versutoxin from the Australian funnel-web spider Hadronyche versuta. Pflugers Arch 428, 400−409.
- Fletcher JI, Chapman BE, Mackay JP, Howden ME & King GF (1997) The structure of versutoxin (delta-atracotoxin-Hvl) provides insights into the binding of site 3 neurotoxins to the voltage-gated sodium channel. Structure 5, 1525−1535.
- Tanaka M, Hainu M, Yasunobu KT & Norton TR (1977) Amino acid sequence of the Anthopleura xanthogrammica heart stimulant, anthopleurin A. Biochemistry 16, 204−208.
- Reimer NS, Yasunobu CL, Yasunobu KT & Norton TR (1985) Amino acid sequence of the Anthopleura xanthogrammica heart stimulant, anthopleurin-B. J Biol Chem 260, 8690−8693.
- Boutjdir M & el-Sherif N (1991) Pharmacological evaluation of early afterdepolarisations induced by sea anemone toxin (ATXII) in dog heart. Cardiovasc Res 25, 815−819.
- Groome J, Lehmann-Horn F & Holzherr B (2011) Open- and closed-state fast inactivation in sodium channels: differential effects of a site-3 anemone toxin. Channels (Austin) 5, 65−78.
- Gomez MV, Kalapothakis E, Guatimosim C & Prado MA (2002) Phoneutria nigriventer venom: a cocktail of toxins that affect ion channels. Cell Mol Neurobiol 22, 579−588.
- West PJ, Bulaj G & Yoshikami D (2005) Effects of delta-conotoxins PVIA and SVIE on sodium channels in the amphibian sympathetic nervous system. J Neurophysiol 94, 3916−3924.
- Leipold E, Hansel A, Olivera BM, Terlau H & Heinemann SH (2005) Molecular interaction of delta-conotoxins with voltage-gated sodium channels. FEBSLett 519, 3881−3884.
- Hitmi A, Coudret A & Barthomeuf C (2000) The production of pyrethrins by plant cell and tissue cultures of Chrysanthemum cinerariaefolium and Tagetes species. Crit Rev Biochem Mol Biol 35, 317−337.
- NobelPrize.org (1948) The Nobel Prize in Physiology of Medicine.
- Soderlund DM (2008) Pyrethroids, knockdown resistance and sodium channels. Pest Manag Sci 64, 610−616.
- Davies TG, Field LM, Usherwood PN & Williamson MS (2007) DDT, pyrethrins, pyrethroids and insect sodium channels. IUBMB Life 59, 151−162.
- Clark JM & Symington SB (2012) Advances in the mode of action of pyrethroids. Top Curr Chem 314, 49−72.
- Jeglitsch G, Rein K, Baden DG & Adams DJ (1998) Brevetoxin-3 (PbTx-3) and its derivatives modulate single tetrodotoxin-sensitive sodium channels in rat sensory neurons. J Pharmacol Exp Ther 284, 516−525.
- Yasumoto T (2001) The chemistry and biological function of natural marine toxins. Chem Rec 1, 228−242.
- Trainer VL, Thomsen WJ, Catterall WA & Baden DG (1991) Photoaffinity labeling of the brevetoxin receptor on sodium channels in rat brain synaptosomes. Mol Pharmacol 40, 988−994.
- Lombet A, Bidard JN & Lazdunski M (1987) Ciguatoxin and brevetoxins share a common receptor site on the neuronal voltage-dependent Na+ channel. FEBS Lett 219, 355−359.
- Benoit E, Legrand AM & Dubois JM (1986) Effects of ciguatoxin on current and voltage clamped frog myelinated nerve fibre. Toxicon 24, 357−364.
- Huang JM, Wu CH & Baden DG (1984) Depolarizing action of a red-tide dinoflagellate brevetoxin on axonal membranes. J Pharmacol Exp Ther 229, 615 621.
- Vassilevski AA, Kozlov SA, Egorov TA & Grishin EV (2010) Purification and characterization of biologically active peptides from spider venoms. Methods MolBiol 615, 87−100.
- Hagiwara K, Sakai T, Miwa A, Kawai N & Nakajima T (1990) Complete amino acid sequence of a new type of neurotoxin from the venom of the spider, Agelena opulenta. Biomed Res 11, 181−186.
- Dilly S, Lamy C, Marrion NY, Liegeois JF & Seutin V (2011) Ion-channel modulators: more diversity than previously thought. Chembiochem 12, 1808−1812.
- Corzo G, Sabo JK, Bosmans F, Billen B, Villegas E, Tytgat J & Norton RS (2007) Solution structure and alanine scan of a spider toxin that affects the activation of mammalian voltage-gated sodium channels. J Biol Chem 282, 46 434 652.
- Cohen C., Bale T., Ertel E., Warren V. & (1993) fx-Aga-IV: A spider toxin specific for insect Na channels. Biophys J 64, A4.
- Norris T., Lee D. & M. A (1995) Modulation of sodium channels by insect-selective scorpion and spider toxins. Soc Neurosci Abstr 21, 1820.