Функциональный анализ генов, кодирующих пептидазы у цианобактерии Synechocystis Sp. PCC 6803
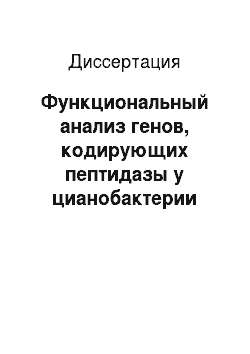
Оксигенный фотосинтез, который осуществляют высшие растения, эукариотические водоросли и прокариотические цианобактерии, является не только единственным глобальным процессом образования кислорода на Земле, но и наиболее эффективным способом биоконверсии солнечной энергии в энергию химических связей. Искусственное воспроизведение процесса фотосинтеза позволило бы создать экологически чистые… Читать ещё >
Содержание
- ВВЕДЕНИЕ
- ОБЗОР ЛИТЕРАТУРЫ
- 1. Цианобактерии как модельные объекты молекулярной генетики и генетической инженерии
- 2. Пептидазы прокариот
- 2. 1. Классификация пептидаз
- 2. 2. АТФ-зависимые пептидазы Escherichia col
- 2. 2. 1. FtsH-металлопептидаза
- 2. 2. 2. Сериновая La/Lon — пептидаза
- 2. 2. 3. Казеинолитические пептидазы (С1р) 2.2.3.1 Пептидаза ClpAP/ClpXP 2.2.3.2. Другие белки С1р-семейства
- 2. 3. Пептидазы Htr-семейства Е. col
- 2. 4. Аминопептидазы и карбоксил-терминальные процессирующие пептидазы
- 3. Генетический контроль синтеза пептидаз у фотосинтезирующих организмов
- МАТЕРИАЛЫ И МЕТОДЫ
- 1. Штаммы бактерий и плазмиды
- 2. Среды и культивирование бактерий
- 3. Трансформация клеток Е. coli плазмидными ДНК
- 4. Трансформация клеток Synechocystis
- 5. Сегрегация трансформантов цианобактерии Synechocystis
- 6. Выделение хромосомной ДНК из Synechocystis
- 7. Выделение плазмидной ДНК из клеток Е. coli кипячением
- 8. Очистка плазмидных ДНК
- 9. Электрофорез ДНК в агарозном геле
- Ю.Элюция фрагментов ДНК из агарозного геля
- 11. Полимеразная цепная реакция (ПЦР)
- 12. Очистка ПЦР фрагментов
- 13. Обработка ДНК эндонуклеазами рестрикции
- 14. «Затупление» выступающих концов ДНК для последующего лигирования
- 15. Лигирование ДНК
- 16. Индуцирование хлороза у цианобактерии
- 17. Определение содержания фотосинтетических пигментов
- 18. Измерение выделения и поглощения кислорода
- 19. Конъюгационные скрещивания
- 20. Определение чувствительности клеток к ингибиторам РЕЗУЛЬТАТЫ И ОБСУЖДЕНИЕ
- 1. Получение инсерционных мутантов ^упескосуяИя
- 2. Физиолого-биохимический анализ инсерционных мутантов по пептидазным генам ШоА, ШоВ, рерР и утхО
- 3. Свойства мутанта, дефектного по пептидазе С1рР
- ВЫВОДЫ
Функциональный анализ генов, кодирующих пептидазы у цианобактерии Synechocystis Sp. PCC 6803 (реферат, курсовая, диплом, контрольная)
Оксигенный фотосинтез, который осуществляют высшие растения, эукариотические водоросли и прокариотические цианобактерии, является не только единственным глобальным процессом образования кислорода на Земле, но и наиболее эффективным способом биоконверсии солнечной энергии в энергию химических связей. Искусственное воспроизведение процесса фотосинтеза позволило бы создать экологически чистые и практически неиссякаемые источники энергии. В связи с этим изучение структуры и функции аппарата фотосинтеза имеет не только фундаментальное, но и прикладное значение.
Удобной моделью для изучения генетического контроля и механизмов фотосинтеза являются цианобактерии, фотосинтетический аппарат которых имеет много общего с системами высших растений, а ключевые гены контролирующие фотосинтез, у обеих групп организмов в значительной степени гомологичны. Таким образом, цианобактерии можно использовать для моделирования процессов фотосинтеза, происходящих в клетках эукариотических организмов. Способность ряда штаммов цианобактерий к генетической трансформации дает широкие возможности для использования методов клонирования генов (Shestakov and Reaston, 1987), а наличие фотогетеротрофных штаммов позволяет выделять и исследовать фотосинтетические мутанты, у которых нарушен процесс фотосинтеза.
С другой стороны, необходимо отметить важное преимущество цианобактерии Synechocystis sp. РСС 6803 (далее обозначаемой как Synechocystis 6803), которое заключается в том, что хромосомная ДНК этого объекта полностью секвенирована (Kaneko et al., 1996, Kotani and Tabata, 1998). Это позволяет находить в банке данных нужные последовательности ДНК, амплифицировать и клонировать их для проведения различных генно-инженерных манипуляций. С помощью этих методов можно проводить 6 инсерционный мутагенез исследуемых генов, инактивируя ген путем встраивания в клонированный фрагмент кассеты устойчивости к антибиотику — то есть использовать приемы «обратной генетики» — от гена к фенотипу.
В исследованиях генетической регуляции фотосинтетического аппарата в последнее время уделяется особое внимание изучению функций генов, кодирующих белки, ответственные за разнообразные процессы протеолиза. У цианобактерии Synechocystis 6803 насчитывается около 60 открытых рамок считывания, которые предположительно кодируют пептидазы (Kaneko et al, 1996, Rawlings and Barrett, 1999). Протеолиз вовлечен в системы клеточного «домашнего хозяйства», в реакции ответа на действие стрессовых факторов путем устранения аномальных белков, в обеспечение аминокислотами процессов синтеза новых белков. Протеолиз необходим для регуляции уровня ключевых ферментов и процессинга предшественников функциональных белков, в том числе при их транслокации через мембрану. Пептидазы играют также значительную роль в процессах созревания и сборки • белков в качестве молекулярных шаперонов (Clarke et al., 1996). К настоящему времени накоплено достаточно доказательств того, что деградация белков — это комплексный процесс, объединяющий множество протеолитических реакций в различных клеточных компартментах. Большинство из пептидаз являются консервативными и обнаруживают гомологию у различных организмов. В тоже время физиологические функции многих из этих пептидаз еще не.
-¦J— идентифицированы. В частности, мало известно о роли конкретных пептидаз в обеспечении и регуляции процессов фотосинтеза. Поэтому молекулярно-генетический анализ генов, кодирующих пептидазы является актуальной проблемой генетики фотосинтезирующих организмов.
Данная работа посвящена молекулярному и функциональному анализу пептидазных генов цианобактерии Synechocystis 6803, как модельного объекта изучения фотосинтеза. 7.
В задачи исследования входило:
1. Получение инсерционных мутантов по генам, кодирующих пептидазы.
2. Изучение физиолого-биохимических характеристик мутантов.
3. Изучение возможной роли пептидаз в регуляции процессов фотосинтеза.
Работа проводилась в рамках международного проекта «Молекулярный анализ генов, участвующих в регуляции фотосинтетической конверсии энергии» по программе «Human Frontier Science Program». 8.
ОБЗОР ЛИТЕРАТУРЫ.
111 выводы.
1. Осуществлена инсерционная инактивация 20 генов, кодирующих различные пептидазы у цианобактерии ЗупескосузШ 6803. Получены гомозиготные мутанты по генам с1рВ2, с1рР2, ргр1, ргр2, ргрЗ, утхС, рерЫ, рерР, рддЕ, ШоА, ШоВ, яррА1, яррА2.
2. Инсерционные мутации в генах с1рВ1, с1рР1, с1рРЗ, с1рР4, с1рХ, згр, ЫгА являются летальными, что свидетельствует о жизненно-необходимых функциях этих генов. Мутанты с дефектными генами с1рВ2, ргр1, ргр2, ргрЗ, рддЕ, утхО, рерЫ, ШоА, ШоВ, яррА1, sppA2 не имеют видимых фенотипических отличий от штамма дикого типа. По-видимому, функции этих генов не проявляются в стандартных фотоавтотрофных условиях роста.
3. Мутант по аминопептидазному гену рерР отличается пониженной скоростью роста в фотоавтотрофных условиях и высокой чувствительностью к индукторам окислительного стресса.
4. Инактивация генов ШоА и ШоВ повышает чувствительность клеток к тепловому шоку, что указывает на возможное участие белков ШоА и НЬоВ в системах адаптации цианобактерии к высокой температуре.
5. Мутант по гену с1рР2 не растет в фотоавтотрофных условиях, но обладает способностью к фотосинтезу. Мутант характеризуется высокой светочувствительностью, что позволяет предполагать участие белка С1рР2 в защите клеток цианобактерии от фотоинактивации.
Список литературы
- Еланская И.В., Бибикова М. В., Богданова С. А., Бабыкин М. М., Шестаков С. В. (1987) Бирепликонные векторные плазмиды для цианобактерии Synechocystis 6803. Докл. АН СССР, 293, 716−719.
- Локшина Л. А. Былинкина B.C. (1990) Протеолитические ферменты в процессинге белков. Успехи современной биологии. М. 109, 219−237.
- Маниатис Т., Фрич Э., Сэмбрук Дж. (1984) Методы генетической инженерии. Молекулярное клонирование. М.: Мир, 480 с.
- Миллер Дж. (1976) Эксперименты в молекулярной генетике. М.: Мир, 436с.
- Шестаков С.В., Кокшарова О. А., Грошев В. В., Еланская И. В. (1988) Дефектные по фотосинтезу мутанты цианобактерии Synechocystis 6803. Биол. науки. № 1. С. 75−80.
- Adam Z. (1996) Protein stability and degradation in chloroplasts. Plant Mol. Biol., 32, 773−783.
- Adamska I., Kloppstech K., and Ohad I. (1993) Early light-induced protein in pea is stable during light stress but is degraded during recovery at low intensity. J. Biol. Chem., 268, 5438−5444.
- Adamska I., Lindahl M., Roobol-Boza M., and Andersson B. (1996) Degradation of the light stress protein is mediated by ATP-independent, serin-type protease under low-light conditions. Eur. J. Biochem., 236, 591−599.
- Aizenman E., Engelberg-Kulka H., and Glaser G. (1996) An Escherichia coli chromosomal 'addiction module' regulated by 3', 5'-bispyrophosphate: a model for programmed bacterial cell death. Proc. Natl. Acad. Sci. USA, 93, 6059−6063.113
- Altschul S.E., Gish W., Miller W., Myers E.W., and Lipman D.J. (1990) Basic local alignment search tool. J. Mol. Biol., 215,403−410.
- Altschul S. F., Madden T. L., Schaffer A. A., Zhang J., Zhang Z., Miller W., and Lipman D. J. (1997) Gapped BLAST and PSI-BLAST: a new generation of protein database search programs. Nucleic Acids Res., 25, 3389−3402.
- Anbudurai P.A., Mor T.S., Ohad I., Shestakov S.V., and Pakrasi H.B. (1994) The ctpA gene encodes the C-terminal processing protease for the D1 of the photosystem II reaction center complex. Proc. Natl. Acad. Sci. USA, 91, 8082−8086.
- Anderson J.M. (1986) Photoregulation of the composition function and structure of thylakoid membranes. Annu. Rev. Plant Physiol., 37, 93−136.
- Andersson B. (1992) Thylakoid membrane dynamic: and stress adaptation. In Trends in Photosynthesis Research (ed. J. Barer, M.G. Guerrero, and H. Medrano), 71−86.
- Andersson B., and Barber J. (1996) Mechanisms of photodamage and protein degradation during photoinhibition of photosystem II. (ed. Baker N.R.) Photosynthesis and the Environment. Kluwer Acad. Publ ., 101−121.
- Archer E.K., and Keegstra K. (1993) Analysis of chloroplast transit peptide function using mutation in the carboxyl-terminal region. Plant Mol. Biol., 23, 11 051 115.
- Aro E.M., Virgin I., and Andersson B. (1993) Photoinhibition of photosystem II. Inactivation, protein damage and turnover. Biochim. Biophys. Acta, 1143,113−134.
- Astier C., Elmorjanik K., Joset F., and Herdman M., (1984) Photosynthetic mutants of the cyanobacterium Synechocystis sp. strain PCC 6714 and PCC 6803: sodium p-hydroxymercuribenzoate as a selective agent. J. Bacterid., 158, 659−664.114
- Barrett A.J., and Rawlings N.D. (1996) Families and clans of cysteine peptidases. Perspect. Drug Discov. Design, 6,1−11.
- Barrett A.J., Rawlings N.D., and Woessner J.F. (1998) Handbook of Proteolytic Enzymes, Academic Press, Lodon, 215.
- Bartsevich V.V., and Pakrasi H.B. (1997) Molecular identification of a novel protein that regulates biogenesis of photosystem I, a membrane protein complex. J. Biol. Chem., 372,6382−6387.
- Bass S., Gu Q., and Christen A. (1996) Multicopy suppressors of pre mutant Escherichia coli include two HtrA (DegP) protease homologs (HhoAB) DksA, and a truncated RlpA. J. Bacterid., 178,1154−1161.
- Brodsky L.I., Drachev A.L., Gorbalenya A.E., Leontovich A.M., and Feranchuk S.I. (1993) A novel method of multiple alignment of bio-polymers (MA-Tools module of GeneBee package). Biosystems, 30, 65−79.
- Brodsky L.I., Ivanov V.V., Kalaydzidis Ya.L., Leontovich A.M., Nikolaev V.K., Feranchuk S.I., and Drachev V.A. (1995) GeneBee-NET: Internet-based server for analyzing biopolymers structure. Biochem., 60, 923−928.
- Bryant D.A. (1991) Photosystem I: polypeptide subunits, genes and mutants. In: Current Topics in photosynthesis, ed Barber J., Elsevier, Amsterdam., 11,432.115
- Burns E.R., Buchanan G. A, and Carter M.D. (1971). Ingibition of carotenoid synthesis as a mechanism of action of amitrole, dichlormate and pyriclor. Plant Physiol., 47,144−148.
- Campbell W.S., and Laudenbach D.E. (1994) Characterization of four superoxide dismutase genes from a filamentous cyanobacterium. J. Bacterid., 177, 964−972.
- Celerin M., Gilpin A.A., Schisler N .J., Ivanov A.G., Miskiewicz E., Krol M., and Laudenbach D.E. (1998) ClpB in a cyanobacterium: predicted structure, phylogenetic relationships, and regulation by light and temperature. J. Bacterid., 180,5173−5182.
- Chitnis P., Reilly P., and Nelson N. (1989) Insertional inactivation of the gene encoding subunit II of photosystem I from the cyanobacterium Synechocystis sp. PCC 6803. J. Biol. Chem., 264, 18 381−18 385.
- Chuang S.E., Burland V, Plunkett G.D., Daniels D.L., and Blattner F.R. (1993) Sequence analysis of four new heat-shock genes constituting the hslTS/ibpAB and /?s7Ft/operons in Escherichia coli. Gene, 134,1−6.
- Clarke A.K. (1999) ATP-dependent Clp proteases in photosynthetic organisms -a cut above the rest! Annals of Botany, 83, 593−599.
- Clarke A.K. (1996) Variation on a theme: combined molecular chaperone and proteolysis functions in Clp/HsplOO proteins. J. Biosci., 21, 166−167.
- Clarke A.K., and Eriksson M-J. (1996) The cyanobacterium Synechococcus sp. PCC 7942 possesses a close homologue to the chloroplast ClpC protein of higher plants. Plant Mol. Biol., 31,721−730.
- Clarke A.K., Gustafsson P., and Lidholm J.A. (1996) Identification and expression of the chloroplast clpP gene in the conifer Pinus contorta. Plant Mol. Biol, 26, 851−862.116
- Clarke A.K., Schelin J., and Porankiewicz J. (1998) Inactivation of the clpPl gene for the proteolytic subunit of the ATP-dependent Clp protease in the cyanobacterium Synechococcus limits growth and light acclimation. Plant Mol. Biol., 37,798−801.
- Collier J.L., and Grossman A.R. (1992) Chlorosis induced by nutrient deprivation in Synechococcus sp strain PCC 7942: Not all bleaching is the same. J Bacterid., 174,4718−4726.
- Cserzo M., Wallin E., Simon I., von Heijne G., and Elofsson A. (1997) Prediction of transmembrane alpha-helices in prokaryotic membrane proteins: the Dense Alignment Surface method. Prot. Eng., 10, 673−676.
- Dagert M., and Ehrlich S.D. (1979) Prolonged incubation in calcium chloride improves the competence of Escherichia coli cells. Gene, 6, 23−28.
- Damerau K., and John A.S. (1993) Role of Clp protease subunits in degradation of carbon starvation proteins in Escherichia coli. J. Bacterid., 175, 53−63.
- Dannehl H., Herbik A., and Godde G. (1995) Stress-indused degradation of the photosynthetic apparatus is accomplanied by changes in thylakoid protein turnover and phosphorylation. Plant Physiol., 93,179−186.117
- Dayhoff M.O., Schwartz R.M., and Orcutt B.C. (1979) A model of evolutionary change in proteins. 345−351 in Atlas of protein sequence and structure, 5, suppl. 3, 1978. National Biomedical Research Foundation, Washington, DC.
- Dzelzkans V.A., and Bogorad L. (1989) Spectral properties and composition of reaction center and ancillary polypeptide complexes of photosystem II deficient mutants of Synechocystis 6803. Plant Physiol., 90,1021−1028.
- Eriksson M-J., and Clarke A.K. (1996) The heat shock protein ClpB mediates the development of thermotolerance in the cyanobacterium Synechococcus sp. strain PCC 7942. J. Bacteriol., 178,4839−4846.
- Farr S.B., and Kogoma T. (1991) Oxidative stress responses in Escherichia coli and Salmonella typhimurium. Microbiol. Rev., 55, 561−585.
- Gavel Y., and Von Heijne G. (1990) A conserved cleavage-site motif in chloroplast transit peptides. FEBS Lett., 261, 455−458.118
- Goff S. A., Casson L.P. and Goldberg A.L. (1984) Heat shock regulatory gene htpR influences rates of protein degradation and expression of the Ion gene in Escherichia coli. Proc. Natl. Acad. Sci. USA, 81, 6647−6651.
- Goldberg A.L. (1992) The mechanism and functions of ATP-dependent proteases in bacterial and animal cells. Eur. J. Biochem., 203,9−23.
- Gottesman S., Clark W.P., de Crecy-Lagard V., and Maurizi M.R. (1993) ClpX, an alternative subunit for the ATP-dependent Clp protease of Escherichia coli. J. Biol. Chem., 268,22 618−22 626.
- Gottesman S., and Maurizi M.R. (1992) Regulation by proteolysis: energy-dependent proteases and their targets. Microbiol. Rev., 56, 592−621.
- Grigorieva G., and Shestakov S. (1982) Transformation in cyanobacterium Synechocystis sp. 6803. FEMS Microbiol. Letters, 13, 367−370.
- Hageman J., Robinson C., Smeekens S., and Weisbeek P. (1986) A thylakoid processing protease is required for complete maturation of the lumen protein plastocyanin. Nature, 324,567−569.
- Hancock J. M., and Armstrong J. S. (1994) SIMPLE34: an improved and enhanced implementation for VAX and Sun computers of the SIMPLE algorithm for analysis of clustered repetitive motifs in nucleotide sequences. Comput. Appl. Biosci., 10, 67−70.
- Hayes S.A., and Dice J.F. (1996) Roles of molecular chaperones in protein degradation. J. Cell Biol., 132, 255−258.119
- Herman C., Thevenet D., D’Ari R., and Bouloc P. (1995) Degradation of a32, the heat shock regulator in Escherichia coli, is governed by HflB. Genetics., 92, 35 163 520.
- Hihara Y., Sonoike K., and Ikeuchi M. (1998) A novel gene pmgA, specifically regulates photosystem stoichiometry in the cyanobacterium Synechocystis species PCC 6803 in response to high light. Plant Physiol., 117,1205−1216.
- Kaneko T., and Tabata S. (1997) Complete genome structure of the unicellular cyanobacterium Synechocystis sp. PCC 6803. Plant Cell. Physiol., 38,1171−1176.120
- Karnauchov I, Herrmann R. G, Pakrasi H. B, and Klosgen R.B. (1997) Transport of CtpA protein from the cyanobacterium Synechocystis 6803 across the thylakoid membrane in chloroplasts. Eur. J. Biochem., 249,497−504.
- Kihara A., Akiyama Y, and Ito K. (1995) FtsH is required for proteolytic elimination of uncomplexed forms of SecY, an essential protein translocase subunit. Cell Biol., 92,4532−4536.
- Kile D. J, Ohad I, and Arntzen C.J. (1984) Membrane protein damage and repair: selective loss of a quinone-protein function in chloroplast membranes. Proc. Natl. Acad. Sci. USA, 81,4070−4074.
- Kotani H, and Tabata S. (1998) Lessons from sequencing of the genome of a unicellular cyanobacterium, Synechocystis sp. PCC6803 Annu. Rev. Plant Physiol. Plant Mol. Biol, 49,151−171.121
- Koussevitzky S., Ne’eman E., Sommer A., Steffens J. C., and Harel E. (1988) Purification and properties of a novel chloroplast stromal peptidase. Processing of polyphenol oxidase and other imported precursors. J. Biol. Chem., 273, 2 706 427 069.
- Krinsky N.I., and Deneke S.M. (1982) Interaction of oxygen and oxy-radicals with carotenoids. J. Natl. Cancer Inst., 69,205−210.
- Kroh H.E., and Simon L.D. (1990) The ClpP component of Clp protease is the sigma 32-dependent heat shock protein F21.5. J. Bacterid., 172, 6026−6034.
- Kruger E., Volker U., and Hecker M. (1994) Stress induction of clpC in Bacillus subtilis and its involvement in stress-tolerance. J. Bacteriol., 6, 3360−3367.
- Kyte J., and Doolittle R. F. (1982) A simple method for displaying the hydropathic character of a protein. J. Mol. Biol., 157,105−132.
- Labarre J., Chauvat F., and Thuriaux P. (1989) Insertional mutagenesis by random cloning of antibiotic resistance genes into the genome of the cyanobacterium Synechocystis strain PCC 6803. J. Bacteriol., 171, 3449−3457.
- Laskowska E., Kuczynska-Wisnik D., Skorko-Glonek J., and Taylor A. (1996) Degradation by proteases Lon, Clp and Htr, of E. coli proteins aggregation in vivo by heat shock, HtrA protease action in vivo and in vitro. Mol. Microbiol., 22, 555 571.
- Levy M., and Adam Z. (1995) Mutations in the processing site of the precursor of ribulose-l, 5-bisphosphate carboxylase oxygenase small subunit: effects on import, processing, assembly and stability. Plant Mol. Biol., 29, 53−61.
- Lichtenthaler H.R. (1987) Chlorophylls and caratenoids: pigments of photosynthetic biomembranes. Methods Enzymol., 148, 350−382.122
- Lindahl M., Yang D.H., and Andersson B. (1995) Regulatory proteolysis of the major light-harvesting chlorophyll a/b protein of photosystem II by a light-induced membrane-associated enzyme system. Eur. J. Biochem., 231, 503−509.
- Lipinska B., Sharma S., and Georgopoulos C. (1988) Sequence analysis and regulation of the htrA gene of Escherichia coir, a sigma 32-independent mechanism of heat-inducible transcription. Nucleic Acids Res., 16,10 053−10 067.
- Lipinska B., Zilick M., and Georgopoulos C. (1990) The HtrA (DegP) protein, essential for Escherichia coli survival at high temperatures, is an endopeptidase. J. Bacterid., 172,1791−1797.
- Liu X-Q., and Jagendorf A.T. (1984) ATP-dependent proteolysis in pea chloroplast. FEBS Letters, 166,248−252.
- Lysenko E., Ogura T., and Cutting S.M. (1997) Characterization of the ftsH gene of Bacillus subtilis. Microbiol., 143, 971−978.
- Malek L., Bogorad L., Ayers A.R., and Goldberg A.L. (1984) Newly synthesized proteins are degraded by an ATP-stimulated proteolytic process in isolated pea chloroplasts. FEBS Letters, 166, 253−257.
- Mannan R.M., and Pakrasi H.B. (1993) Dark heterotrophic growth conditions result in an increase in the content of photosystem II units in the filamentous cyanobacterium Anabaena variabilis ATCC 29 413. Plant Physiol., 103, 971−977.
- Marder J.B., Goloubinoff P., and Edelman M. (1984) Molecular architecture of rapidly metabolized 32-kilodalton protein of photosystem II: indications for COOH-terminal processing of a chloroplast membrane polypeptide. J. Biol. Chem., 259, 3900−3908.
- Maurizi M.R. (1991) ATP-promoted interaction between ClpA and ClpP in activation of Clp protease from Escherichia coli. Biochem. Soc. Trans., 19, 719−723.123
- Maurizi M.R. (1992) Proteases and protein degradation in Escherichia coli. Experentia, 48, 178−201.
- Maurizi M.R., Clark W.P., Katayama Y., Rudikoff S., and Pumphrey J. (1990a) Sequence and structure of ClpP, the proteolytic component of the ATP-dependent Clp protease of Escherichia coli. J. Biol. Chem., 265,12 536−12 545.
- Maurizi M.R., Clark W.P., Kim S-H., and Gottesman S. (1990b) ClpP represents a unique family of serine proteases. J. Biol. Chem., 265,12 546−12 552.
- Maurizi M.R., Thompson M.W., Singh S.K., and Kim S.H. (1994) Endopeptidase Clp: ATP-dependent Clp protease from Escherichia coli. Methods Enzymol. 244, 14−331.
- Melis A. (1999) Photosystem-II damage and repair cycle in chloroplasts: what modulates the rate of photodamage in vivo? Trends in Plant Sci., 4,130−135.
- Meyer R.J., and Shapiro J.A. (1980) Genetic organization of the broad-host-range IncP-1 plasmidR751. J. Bacterid, 143,1362−1373.
- Mhammedi-Alaoui A., Pato M., Gama M.-J., and Toussaint A. (1994) A new component of bacteriophage Mu replicative transposition machinery: the Escherichia coli ClpX protein. Mol. Microbiol., 11,1109−1116.
- Musgrove J.E., Elderfield P.D., and Robinson C. (1989) Endopeptidases the stroma and thylakoids of pea chloroplasts. Plant Physiol., 90, 1616−1621.
- Nakabayashi K., Ito M., Kiyosue T., Shinozaki K., and Watanabe A. (1999) Identification of clp genes expressed in senescing Arabidopsis leaves. Plant Cell Physiol., 40, 504−514.
- Nakamura Y., Kaneko T., Hirosawa M., Miyajima N., and Tabata S. (1998) CyanoBase, a www database containing the complete nucleotide sequence of the genome of Synechocystis sp. strain PCC 6803. Nucleic Acids Res., 26, 63−67.
- Nikolaev V.K., Leontovich A.M., Drachev V.A., and Brodsky L.I. (1997) Building multiple alignment using iterative analyzing biopolymers structure dynamic improvement of the initial motif alignment. Biochem., 62, 578−582.
- Niyogi K.K. (1999) Photoprotection revisited: genetic and molecular approaches. Annu. Rev. Plant Physiol. Plant Mol. Biol., 50, 333−359.
- Oblong J.E., and Lamppa G.K. (1992) Identification of two structure related proteins involved in proteolytic processing of precursors targeted to the chloroplast. EMBO J., 11,4401−4404.
- Oka A., Sugisaki H., and Takanami M. (1981) Nucleotide sequence of the kanamycin resistance transposon Tn903 J. Mol. Biol., 147,217−226.125
- Packer J.C.L, Andre D, and Howe C.J. (1995) Cloning and sequence analysis of a signal peptidase I from the thermophilic cyanobacterium Phormidium laminosum. Plant Mol. Biol, 27, 199−204.
- Pallen M. J, and Wren B.W. (1997) The HtrA family of serine proteases. Mol. Microbiol, 26, 209−221.
- Parsell D. A, and Lindquist S. (1993). The function of heat-shock proteins in stress tolerance: degradation and reactivation of damaged proteins. Annu. Rev. Genet, 27,437−496.
- Parsell D. A, Sanchez Y, Stitzel J. D, and Lindquist S. (1991) HSP104 is a highly conserved protein with two essential nucleotide-binding sites. Nature, 353, 270−273.
- Parsell D. A, Taulien J, and Lindquist S. (1993) The role of heat-shock proteins in thermotolerance. Philos. Trans. Royal. Soc. London Ser. B, 339, 279−286.
- Pinna L.A. (1990) Casein kinase 2: an 'eminence grise' in cellular regulation. Biochem. Biophys. Acta, 1054,267−284.
- Ponting C.P. (1997) Evidence for PDZ-domains in bacteria, yeast and plants. Protein Sci, 6, 464−468.
- Porankiewicz J, and Clarke A. K, (1997) Induction of the heat shock protein ClpB affects cold acclimation in the cyanobacterium Synechococcus sp. strain PCC 7942. J. Bacterid, 179, 5111−5117.126
- Porankiewicz J., Schelin J., and Clarke A.K. (1998) The ATP-dependent Clp protease is essential for acclimation to UV-B and low temperature in the cyanobacterium Synechococcus. Mol. Microbiol., 29, 275−283.
- Porankiewicz J., Wang J., and Clarke A.K. (1999) New insights into the ATP-dependent Clp protease: Escherichia coli and beyond. Mol. Microbiol., 32,449−458.
- Prasil O., Adir N, and Ohad I. (1992) Dynamics of photosystem II: mechanism of photoinhibition and recovery processes. Topics in Photosynthesis. Structure, Function and Mol. Biol., 11, 295−348.
- Rawlings N.D., and Barrett A.J. (1993) Evolutionary families of peptidases. Biochem. J., 290,205−218.
- Rawlings N.D., and Barrett A.J. (1994a) Families of cysteine peptidases. Methods Enzymol., 244,461−486.
- Rawlings N.D., and Barrett A.J. (1994b) Families of serine peptidases. Methods Enzymol., 244,19−61.
- Rawlings N.D., and Barrett A.J. (1995a) Evolutionary families of metallopeptidases. Methods Enzymol., 248,183−228.
- Rawlings N.D., and Barrett A.J. (1995b) Families of aspartic peptidases, and those of unknown catalytic mechanism. Methods Enzymol., 248,105−120.
- Rawlings N.D., and Barrett A.J. (1997) Classification of peptidases by comparisons of primary and secondary structures. Proteolysis in Cell Functions Eds Hopsu-Havu V.K., Jarvinen M., Kirschke H., IOS Press, Amsterdam, 13−21.
- Rawlings N.D., and Barrett A.J. (1999) MEROPS: the peptidase database. Nucleic Acids Res., 27,325−331.127
- Rep M., and Grivell L.A. (1996) The role of protein degradation in mitochondrial function and biogenesis. Review. Curr. Genet., 30, 367−380.
- Rintamaki E., Kettunen R., Tystjarvi E., and Aro E.M. (1995) Light-dependent phosphorylation of D1 reaction center of photosystem II: hypothesis for the functional role in vivo. Plant Physiol., 93,191−195.
- Rippka R., Deruelles J., Waterbury J.B., and Stanier R.Y. (1979) Genetic assignments, strain histories and properties of pure cultures of cyanobacteria. J. Gen. Microbiol., Ill, 1−61.
- Robinson C., and Ellis R.J. (1984) Transport of protein into chloroplasts: partial purification of a chloroplast protease involved in the processing of imported precursor polypeptides. Eur. J. Biochem., 142, 337−342.
- Robinson C., and Klosgen R.B. (1994) Targeting of proteins into and across the thylakoid membrane: a multitude of mechanisms. Plant Mol. Biol., 26,15−24.
- Rose R.E. (1988) The nucleotide sequence of pACYC184. Nucleic Acids Res., 16, 355.128
- Sakamoto T., and Bryant D.A. (1998) Growth at low temperature sauses nitrogen limitation in the cyanobacterium Synechococcus sp. PCC 7002. Arch. Microbiol., 169,10−19.
- Schirmer E.C., Glover J.R., Singer, M.A., and Lindquist S. (1996) HSPlOO/Clp proteins: a common mechanism explains diverse functions. TIBS, 21, 289−296.
- Schroda M., Vallon O., Wollman F-A., and Beck C.F. (1999) A chloroplast-targeted heat shock protein 70 (HSP70) contributes to the photoprotection and repair of photosystem II during and after photoinhibition. Plant Cell, 11,1165−1178.
- Schweder T., Lee K.H., Lomovskaya O., and Matin A. (1996) Regulation of Escherichia coli starvation sigma factor (as) by ClpXP protease. J. Bacterid., 178, 470−476.
- Schweirer H.P. (1993) Small broad-host-range gentamycin resistance gene cassettes for site-specific insertion and deletion mutagenesis. Biotechniques, 15, 831−833.
- Shanklin J., DeWitt N.D., and Flanagan J.M. (1995) The stroma of higher plant plastids contain ClpP and ClpA: an archetypal two-component ATP-dependent protease. Plant Cell, 7,1713−1722.
- Shestakov S.V., Anbudurai P R., Stanbekova G.E., Gadzhiev A., Lind L.K., and Pakrasi H.B. (1994) Molecular cloning and characterization of the ctpA gene encoding a carboxyl-terminal processing protease. J. Biol. Chem., 269, 1 935 419 359.
- Shestakov S.V., and Khyen N.T. (1970) Evidence for genetic transformation in blue-green alga Anacystis nidulans. Molecular and General Genetics, 107, 372−375.
- Shestakov S.V., and Reaston J. (1987) Gene transfer and host-vector systems of cyanobacteria. Oxford Surveys of Plant Molecular & Cell Biology, 4,137−166.129
- Skorko-Glonek J., Krzewski K., Lipinska B., Bertoli E., and Tanfani F. (1995a) Comparison of the structure of wild type HtrA heat shock protease and mutant HtrA proteins. J. Biol. Chem., 270,11 140−11 146.
- Smith I.K. (1985) Stimulation of glutathion synthesis in photorespiring plants by catalase inhibitors. Plant Physiol., 79,1044−1047.
- Sokolenko A., Lerbs-Mache S., Altschmied L., and Herrmann R.G. (1998) Clp protease complexes and their diversity in chloroplasts. Planta, 207286−295.
- Songyang Z., Fanning A.S., Fu C., Xu J., Marfatia S.M., Chishti A.H., Crompton A., Chan A.C., Anderson J.M., and Cantley L.C. (1997) Recognition of unique carboxyl-terminal motifs by distinct PDZ domains. Science, 275, 73−77.
- Starke P.E., and Farber J.L. (1985) Endogenous defenses against the cytotoxicity of hydrogen peroxide in cultured rat hepatocytes. J. Biol. Chem., 260, 86−92.
- Stevens S. E., and Porter R. D. (1980) Transformation in Agmenellum quadriplicatum. Proc. Natl. Acad. Sci., USA, 77, 6052−6056.
- Spetea C., Hundal T., Lohmann F., and Andersson B. (1999) GTP bound to chloroplast thylakoid membranes is required for light-induced, multienzyme degradation of the photosystem IID1 protein. Plant Biol., 96, 6547−6552.
- Suzuki C.K., Rep M., Dijl J.V., Suda K., Grivell L.A., and Schatz G. (1997) ATP-dependent proteases that also chaperone protein biogenesis. Reviews. TIBS, 22,118−123.130
- Taylor L. A, and Rose R.E. (1988) A correction in the nucleotide sequence of the Tn903 kanamycin resistance determinant in pUC4K. Nucleic Acids Res, 16, 358−358.
- Tomoyasu T, Gamer J, Bukau B, Kanemori M, Mori H, Rutman A. J, Oppenheim A. B, Yura T, Yamanaka K, and Niki H. (1995) E. coli FtsH is a membrane-bound, ATP-dependent protease which degrades the heat-shock transcription factor 32. EMBO J, 14,2551−2560.
- Tomoyasu T, Yamanaka K, Murata K, Suzaki T, Bouloc P, Kato A, Niki H, Hiraga S, and Ogura T. (1993a) Topology and subcellular localization of FtsH protein in Escherichia coli. J. Bacteriol, 175, 1352−1357.
- Van den Hondel C, and Van Arkel G. (1980) Development of a cloning system in cyanobacteria. Antonie Van Leeuwenhoek, 46, 228−229.
- Wang S, and Liu X-Q. (1997) Identification of an unusual intein in chloroplast clpP protease of Chlamydomonas eugametos. J. Biol. Chem, 272, 11 869−11 873.
- Wickner S., Gottesman S., Skowyra D., Hoskins J., McKenney K., and Maurizi M.R. (1994) A molecular chaperone, ClpA, functions like DnaK and DnaJ. Proc. Natl. Acad. Sci. USA, 91,12 218−12 222.
- Wilce M.C., Bond C.S., Dixon N.E., Freeman H.C., Guss J.M., Lilley P.E., and Wilce J.A. (1998) Structure and mechanism of a proline-specific aminopeptidase from Escherichia coll Proc. Natl. Acad. Sci. USA, 95, 3472−3477.
- Wojtkowiak D., Georgopoulos C. and Zylicz M. (1993) Isolation and characterization of ClpX, a new ATP-dependent specificity component of the Clp protease of Escherichia coli. J. Biol. Chem., 268, 22 609−22 617.
- Woo K.M., Kim K.I., Goldberg A.L., Ha D.B., and Chung C.H. (1992) The heat-shock protein ClpB in Escherichia coli is a protein activated ATPase. J. Biol. Chem., 267, 20 429−20 434.
- Yanisch-Perron C., Viera J., and Messing J. (1985) Improved M13 phage cloning vectors and host strains: nucleotide sequences of the M13mpl8 and pUC19 vectors. Gene, 33,103−119.
- V/169.Zinchenko V.V., Babykin M.M., and Shestakov S.V. (1984) Mobilization of non-conjugative plasmids into Rhodopseudomonas sphaeroides. J. Gen. Microbiol., 130,1587−1590.
- Zinchenko V.V., Piven I.V., Melnik V.A., and Shestakov S.V. (1999) Vectors for the complementation analysis of cyanobacterial mutants. Rus. J. Genet., 35, 228 232.132